By Graham Templeton on May 28, 2013
Back when George W. Bush announced a $1.2 billion plan to advance hydrogen fuel cells, the idea seemed rather implausible. Though it’s the most abundant element in the universe by far, in our environment hydrogen hides so effectively that our methods of drawing it out as a so-called “zero-emissions” fuel have tended to be either ineffective or extremely dirty. Producing hydrogen through the electrolysis of water was something of a holy grail to the hydrogen crowd, but suffered from the same drawbacks as traditional hydrogen sources: we needed to burn coal to make power to make hydrogen to make power. Often, hydrogen power simply traded the emission of airborne carbon at the consumer end for precisely the same emissions at the production end — hardly a great leap forward.
Still, research has gone on. There’s simply too much potential in a fuel source that can already make a rally-level supercar
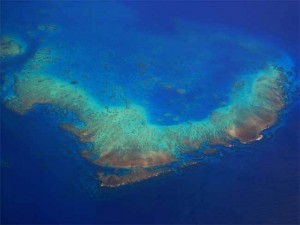
The Great Barrier Reef, which is under attack from ocean acidification
The water left over after we’ve squeezed out the hydrogen fuel is said to be an electrolyte solution with a very high affinity for atmospheric CO2 — a claim that, if true, would be remarkable. As the researchers themselves note, most of the current methods of atmospheric carbon capture are cumbersome, requiring all sorts of energy input. Though there are currently no numbers available to quantify the efficiency of this system, even a moderate ability to take up atmospheric CO2 through the simple interface of air and water would be an incredible step forward.
But the claims don’t stop there! Upon absorbing the atmospheric CO2, the solution becomes saturated with high pH-producing (alkali) molecules like carbonates and bicarbonates, substances they hope could be used in neutralizing ocean acidification. The continual drop in oceanographic pH (increase in acidity) is arguably one of the most worrying effects of atmospheric carbon, as up to 40% of the CO2 released will eventually be dissolved into the world’s oceans, lakes, and rivers. Where atmospheric CO2 works on a rather abstract, global scale, ocean acidification works on the local one as well, devastating or sometimes completely destroying local ecosystems.
While it might seem like a bit of a pipe dream to just dump a bunch of bicarbonate into the sea and hope that everything evens out, the precise reaction the researchers are referring to is actually one of the most common acid-base reactions in all of nature. When CO2 is absorbed by water, it forms carbonic acid, which can be neutralized by carbonates; if these names sound familiar, it’s because the carbonic acid-bicarbonate buffer is the primary acid-base buffer found in life itself. At all times, the interplay between these two types of molecule keeps your blood from becoming either too acidic or too basic.
[4]
A water electrolysis plant. This type of plant currently makes only a tiny minority of the world’s purified hydrogen.
Much is still unknown, since the paper won’t be released in full for another few days. Still, Lawrence Livermore is standing behind the study, and claims to want to ramp up the team’s lab-scale experiments up to see their real-world implications. We need to know just how much energy is required to produce this hydrogen fuel and its carbon-sink water byproduct, and we also need to know precisely how much carbon can really be captured. If, for instance, we were to power 10% of American automobiles with this technology, how much atmospheric carbon would that actually represent? How much usable bicarbonate could then be extracted? And what sort of water are we left with as a waste product after all this has been done?
Regardless, this study is an indication of a growing interest in linking our technologies to help one another, or to at least offset each other’s drawbacks. Forgetting cars for a moment, might we all switch to a hydrogen furnace in our home? Keep in mind that doing so would likely not be a move for personal efficiency, since conservation of energy makes it inherently inefficient to add another conversion between the original energy production and the eventual use of that energy. Rather, it would be a move to help the environment.
If these researchers have their way, we could see our environmentalist initiatives turned on their head: “Save the planet,” the signs would say. “Leave your lights on!”